Emerging magnetism on vibrating non-magnetic layers
Researchers demonstrate that phonons can be used to induce and control magnetic responses in non-magnetic layers
Researchers from the Theory Department of the MPSD (Max Planck Institute for the Structure and Dynamics of Matter) at the Center for Free-Electron Laser Science in Hamburg and UNIST (Ulsan National Institute of Science and Technology) in Korea used state-of-the-art theoretical simulation tools to demonstrate the possibility to induce and control a magnetic response in a non-magnetic layer material though selective excitation of a specific vibration of the material.
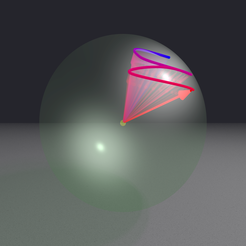
The authors show that circularly polarized phonons produce a valley-dependent dynamic spins state as a result of strong spin-phonon coupling, which can be developed as a vehicle for quantum computation or spintronics applications. Their work, Phonon-driven spin-Floquet magneto-valleytronics in MoS2, is published in Nature Communications.
In everyday life we experience and utilize many properties of materials. These range from electrical and thermal conductors or insulators to micro- / nano-electronics, sensors, telecommunications, computing, energy conversion and tailored materials with specific mechanical, optical and magnetics properties, to name a few. Microscopically, these materials consist of electrons and nuclei, and their properties can mostly be attributed to the quantum mechanical arrangement of the electrons.
Even though the atomic nucleus can also be specified by its constituent particles, such as protons and neutrons, the inner structure of the nucleus usually plays no role in determining the properties of the material. Instead, nuclei are clearly manifested through their vibration. The shape and magnitude of the vibrations, which is specifically called a phonon, are another major factor determining the material’s properties besides the charge and spin of the electrons.
Nowadays researchers focus on elemental structures of materials with the aim of ultimately miniaturizing magnetic units or electronic devices. Graphene, the carbon single layer, and the monolayer of transitional metal dichalcogenide (TMDC) are prime examples in this field. Whether the spins in these two-dimensional materials can be aligned to form a magnet or how sensitively they are affected by the atomic vibration have remained important topics among physicists.
In the current study, taking MoS2 and other TMDCs as sample materials, the team studied how the spin structure can be changed by the presence of a phonon. Extensive supercomputing calculations of quantum mechanical equations showed that, when a material has strong coupling between the spin and orbital state of its electrons, a particular phonon can derive a spin dynamic in a similar way as a rotating magnetic field does.
This effect relies on a fundamental concept of theoretical physics called symmetry breaking. Especially in crystals, the symmetries of the arrangements of atoms play a decisive role, and breaking one of them often brings dramatic changes in the properties of the material.
The symmetry of a system does not only involve space, but can also be extended to time. In what is called time-reversal symmetry, the observed physics would be the same if we go forward or backwards in time. For example, in a video of two colliding billiard balls, you cannot tell whether the video is running forward or backward, because in both cases the physics looks identical.
However, in the presence of a magnetic field, the motion of the electrons cannot be cheated in this way because it does not have symmetry between the forward-moving and backward-moving state. Instead of a magnetic field, many researchers have recently used a circularly polarized photon, or a rotating state of light, to break the time-reversal symmetry.
Instead of a photon, researchers used the rotating state of a phonon, i.e. a circular motion of atoms in a crystal, as a new type of time-reversal breaking mechanism. They showed that such phonons can be compared to the presence of the magnetic field and can be used for the fast manipulation of magnetic units of materials.