Handed light throws electronic curve balls in quantum flatland
An international team of researchers from Switzerland, Germany, and the USA has demonstrated that the Berry curvature - an important property of quantum materials - can be imaged with chiral light. Using advanced theoretical concepts and extensive numerical simulations, the team predicted the outcomes of photoelectron spectroscopy experiments and showed that the resulting images reveal information about the microscopic structure of electronic wavefunctions inside atomically thin, two-dimensional materials. This proposal could lead to high-precision imaging diagnostics of novel materials and pave the way for future quantum technologies employing the Berry curvature of matter. The work has now been published in Science Advances.
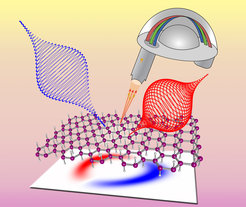
We all live in a curved geometry: the earth is not flat. We realize this whenever we board a plane across the Atlantic Ocean and seemingly take a detour - if viewed on a flat map - via Iceland and Greenland to travel from Europe to the United States. Electrons in a material experience a similar phenomenon. Their motion is affected by a quantity called the Berry curvature, named after Sir Michael Berry, who first gave a comprehensive description of the phenomenon in a hallmark paper in 1984.
The Berry curvature has important consequences, for example in the quantum Hall effect (Nobel Prize 1985), which nowadays sets the standard for the most precise measurements of fundamental constants of nature (electron charge, Planck constant). The concept of the Berry curvature has also revolutionized modern condensed matter physics. It is intimately related to topology, the concept that describes the properties which remain intact when an object is deformed but not torn apart. Nowadays the study of topology in various branches of physics is one of the hot topics in the scientific community.
However, unlike in the case of so-called quantum simulators, where imaging of the Berry curvature is directly possible and has been demonstrated in Hamburg, no such imaging technique has been invented for solid materials. This is where the new results come to the rescue. The team proposes to use photoelectron spectroscopy to measure Berry curvature in solids.
Photoelectron spectroscopy is based on the photoelectric effect. Albert Einstein’s description of this phenomenon earned him the Nobel Prize in 1921. Nowadays, researchers use light that is energetic enough to knock electrons out of a material to measure the so-called band structure -- which determines whether a material is insulating, semiconducting, or metallic. Thus photoelectron spectroscopy is now one of the most important techniques used to investigate the electronic properties of materials.
In the new work, Michael Schüler, formerly a postdoctoral researcher at the University of Fribourg, Switzerland and now based at SLAC and Stanford University, USA, together with Umberto De Giovannini from the Max Planck Institute for the Structure and Dynamics of Matter (MPSD) in Hamburg and colleagues suggest adding a twist to conventional photoelectron spectroscopy. The laser light has an additional property, namely polarization. Circularly polarized light has a handedness -- right-handed or left-handed -- also known as chirality, which means that the electromagnetic field of the light wave rotates clockwise or counter-clockwise as the light moves through space. In their numerical simulations, the researchers showed that the photoelectron signal looks different for right-handed versus left-handed light. Moreover, they showed that this difference is directly tied to the Berry curvature of the electronic wavefunctions inside the material.
"We show that one can tease out more information about the electrons than merely their energy and momentum, which are usually mapped out", explains Schüler. "We have calculated the expected signals for some typical and important atomically flat materials, which are now at the forefront of quantum materials science and seen as potential candidates for quantum technologies", adds De Giovannini. "It is surprising how well this method works and we expect that experimental advances in these imaging techniques will enhance our knowledge about topological properties in the very near future."
The researchers also expect that the technique can be generalized to the ultrafast dynamical regime. "We are at the beginning of a nonequilibrium revolution,"adds Philipp Werner, a physics professor in Fribourg. "There are numerous theory proposals and some very promising first experimental demonstrations of topological properties in materials that are changed on femtosecond time scales by the application of ultrashort laser pulses.” Angel Rubio, theory director at MPSD, predicts: "Our method of imaging topological properties can be directly generalized to this time domain, and will allow important insights into how light can be used to create properties on demand in quantum materials."