Quantum biology revisited: separating fact from friction
Review by 18 leading researchers from 16 institutions in Science Advances demonstrates that inter-exciton coherences are too short-lived to have any functional significance in photosynthetic energy transfer and points to impulsively excited vibrations instead.
The emergence and development of photosynthesis played a key role in the genesis of life on Earth about four billion years ago. This process is as old as life itself and would be expected to be one of the most evolutionarily optimized biological processes in nature. At every level one inspects the different features of photosynthesis, one sees a mastery of details to fully optimize the conversion of solar energy into oxygen, carbohydrates, and stored energy, the “stuff of life”. This optimization occurs at the molecular level, which is the domain of quantum mechanics. At each level of optimization, we see the fingerprints of quantum effects that have been exploited to efficiently direct the absorption of photons, the quantized bits of energy, and its energy transfer process to bring forth life. It is in this regard that one must look to the energy transport process in photosynthesis as one of the most optimized processes of living systems.
Quantum mechanics explicitly treats the wave properties of matter, which requires treating both amplitude and phase in describing the system of interest. The most fundamental difference between quantum mechanics and classical mechanics in describing the dynamics of matter is this notion of phase. It is the relative phase relationship of interacting quantum systems that leads to constructive and destructive interference effects that give rise to “spooky” action at a distance associated with quantum mechanics. Is it possible that nature has so fully optimized processes that even the phase of the underlying matter waves is exploited? If so, one might expect to see evidence for conserved phase relationships or coherence lasting for at least the timescale needed for directing the biological process. This question has been asked since the inception of Quantum Mechanics. The best place to look for such manifestations would be in the most optimized and fastest biological processes to beat out decoherence effects or loss of phase information in the system’s response, namely the energy transport process of photosynthesis.
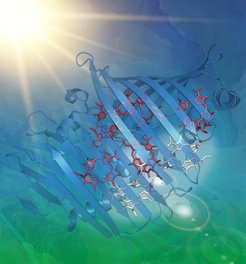
Given the importance of this problem, photosynthesis has been extensively investigated with numerous theoretical and experimental tools over the past three decades. The energy transfer and motion from one subset of chlorophyll molecules to another till it reaches the reaction center has been completely mapped out. The time scale for the transfer of one absorption site to another has been determined to occur on hundred femtosecond (10-13 s) timescales with overall energy transfer time to the reaction center to take several picoseconds (10-12 s), i.e. extremely fast but not as fast as decoherence in the wave properties of this process. The energy transport is viewed within what is called an exciton picture, in which the initial excitation created by the absorption of a photon is partially delocalized to some degree over the various chlorophyll sites. Based on theory and indirect experiments, this coherence in phase relationship between chlorophyll sites was expected to be rapidly lost due to random thermal motion. In this case, the excitation transfer occurs as a series of incoherent hops given as a probability in going from one collective exciton state to another, downhill in energy. This transport process is akin to watching a drunken sailor stumble downhill. This was the established picture for many years that helped explain the efficiency of energy transfer.
With the introduction of femtosecond 2D photon echo methods or 2D spectroscopy, it was possible to directly observe the electronic couplings between chlorophylls or exciton basis, the very interaction giving the probability for the hopping times. This feature shows up in the off diagonal features of 2D spectra much like spin couplings in 2D NMR. We now had the opportunity to directly measure the couplings and coherence time as these off diagonal features will beat at the difference frequency between coupled states as long as the coherence is conserved.
In 2007, there was a paper published in Nature (Engel et al) applying 2D spectroscopy to the Fenna-Matthews-Olsen (FMO) antenna complex. The surprise was the observation of long-lived oscillations in the off diagonal components of the 2D spectra, the components typically associated with the electronic couplings involved in energy transfer. This work and the many papers that followed attributed these small amplitude oscillations or quantum beats observed in this and other systems to conserved quantum coherence in the coupling between chlorophylls. It was asserted that nature was exploiting quantum phase relations to coherently transport energy in a wave like fashion to the reaction center. For this to be true, nature would have discovered how to cancel out thermal noise to conserve the phase and coherently direct the energy to the target site without careening off uncorrelated thermal motions. The prospect that it was possible conserve fragile quantum coherences in a wet complicated noisy environment within photosynthetic light harvesting complexes captured the imagination. If this observation was true, then there would be new means to avoid quantum decoherence effects limiting quantum computing, transfer of quantum information etc. Many more experiments and theory came forward that seemed to support this notion and proposed new mechanisms for conserving quantum coherence. The field effectively exploded.
There was an excitement in the field that we were just beginning to learn from nature how to overcome decoherence even at room temperature in noisy environments to fully control quantum effects. The problem is that the original conjecture was incorrect. Given the importance of photosynthesis, this review now published in Science Advances was motivated to set the science right.
This review article written by 18 leading researchers from 16 institutions in ten countries (among them the MPSD and the Hamburg Cluster of Excellence CUI : Advanced Imaging of Matter), discusses recent work reexamining these claims and demonstrates that inter-exciton coherences are too short-lived to have any functional significance in photosynthetic energy transfer. Instead, the observed long-lived coherences are unambiguously shown to arise from impulsively excited vibrations, which was not considered in the initial publications igniting this interest. The occurrence of small amplitude vibrational coherences is generally observed in femtosecond spectroscopy and needs to be included in the analysis. This review provides a tutorial on the interpretation of 2D spectra and how to separate electronic and vibrational contributions to the spectrum so that readers outside the area can critically evaluate evidence given for coherent effects and their origins. The review also highlights the major improvements in theoretical and experimental methods that have led to a deeper understanding of the system-bath interactions responsible for decoherence and dissipation in biological systems.
The take home message is that nature does not engineer the bath to avoid decoherence to direct functions; such an approach would certainly not be robust to noise or random changes in phase. Rather than trying to avoid dissipation processes, or molecular friction, that leads to quantum decoherence, nature specifically exploits it by engineering site energies of chlorophylls to create a downhill energy pathway to direct energy transport. It is well appreciated that downhill relaxation processes are used to entropically drive biological functions on other levels of living systems. This review points out that this principle applies even to the energy transfer processes involved in photosynthesis that occur on the fastest possible timescales. The basic physics behind thermalization is used to impose direction. In the words of R. J. Dwayne Miller, the director of the Atomically Resolved Dynamics department at the MPSD: “This simple concept, mastered by nature over all relevant time and spatial dimensions, rather than esoteric quantum effects, is the true marvel of biology.”