Electrons: collective or independent – that sometimes lies in the eye of the beholder
The absorption of light adds energy to a material. The electrons inside the material can react collectively or independently to that energy. Here, the dominant reaction pattern should not depend on perspective. However, in compound semiconductors, surprisingly, divergent responses are obtained depending on which atomic constituent is consulted. Knowledge of both perspectives is necessary to optimize new materials for applications in electronics and optoelectronics.
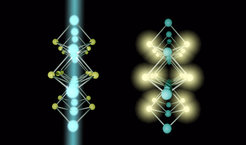
The electronic properties of semiconductor materials composed of different chemical elements, so-called compound semiconductors, result from their specific combination of atomic species. It is the bonds between these atoms that determine the overall properties of the material and not the atomic species themselves.
Unexpectedly, researchers at the Department of Physics at ETH Zurich received contradictory answers when they investigated how the electrons of the compound semiconductor MoSe2 behave within a few femtoseconds (1 femtosecond = 10-15 s) after absorbing a short pulse of infrared light [1]. The respective responses depended on whether the measurement took place via Mo or via Se atoms.
When a material absorbs light, the electrons as its lightest components react first. As a result, various processes can take place. For example, the electrons can assume previously unoccupied energy states and release their previously occupied places. In this process, the electrons act independently of each other. Or they gather more or less around the positive atomic nuclei and influence how these positive charges act on the totality of electrons. Cause and effect of this process is only understandable if the electrons are considered collectively.
Whether the independent or collective processes dominate depends on the material. For example, in transition metals the collective effects are particularly important [2], while in simpler metals (such as aluminum) the electrons can be considered independent [3]. In the same way, in semiconductors, processes usually dominate in which the interaction between electrons does not take an important role.
How does one now investigate the reaction of the electrons to the absorption of infrared light? Only femtoseconds after the impact of the infrared pulse, a second light pulse in the extreme ultraviolet is sent to the sample. Ultraviolet photons have very high energy. Thus, the second pulse can now carry electrons from low-lying atomic energy levels into the important energy region of interatomic bonds.
If the preceding infrared pulse has removed an electron from an energy state, space is now freed up for the electron excited by the ultraviolet pulse. This can be seen in experiment by an increased absorption of extreme ultraviolet light in a characteristic spectral region. Similarly, the collective processes also cause a characteristic absorption change for the ultraviolet pulse.
Zeno Schumacher, a former postdoc at the Institute for Quantum Electronics at ETH Zurich, and his co-authors - including Shunsuke Sato and Angel Rubio from the MPSD‘s Theory Department - used the fact that they were able to observe the energy region of the bonds in the compound semiconductor MoSe2 via low-lying energy levels of the Mo as well as the Se atoms, simultaneously. The spectrally very broadband ultraviolet pulses made it possible to separate the signals from the two atomic species in energy.
To the great surprise of the study‘s authors, the Mo signal clearly showed a signature dominated by collective processes. In contrast, the Se signal suggested that the electrons in this material act independently. Even though the electronic properties of MoSe2 are determined by the bonds of Mo and Se atoms, to which both elements contribute equally, the material shows opposite faces depending on through which of its atomic constituents it is probed.
Despite the material MoSe2 being a semiconductor it displays the collective effects already known from pure transition metals [2]. The authors from ETH Zurich and the Max Planck Institute for the Structure and Dynamics of Matter conclude from theoretical considerations that this should also be the case in other compounds of transition metals and nonmetals.
In similar experiments in the past, often only the signal from one involved atomic species was considered. However, the new study shows that one perspective is not sufficient to understand the behavior of the complete system. Both "faces" of MoSe2 are an integral part of its electronic character.
In semiconductor theory today, electrons are usually described very successfully under the assumption of a so-called effective mass. The effective mass combines the effects of the environment of the electrons on their motion under the influence of external forces into a correction of their mass. However, the concept of an effective mass implies that electrons move independently. If nothing else, the work presented here shows that this is only an incomplete description of their behavior.
A common criticism of similar laser-based studies is that the conditions to which the material is exposed are not comparable to interactions with light from more commonplace sources, such as sunlight or light from light-emitting diodes or incandescent bulbs. However, the theoretical analysis presented by the authors shows that the experiment can be explained by a simple increase in electron temperature brought about by the infrared light. From this, they conclude that their results should also be applicable to less intense light sources. This is relevant because the family of compound semiconductors to which MoSe2 belongs holds strong promise for future applications in optoelectronics and electronics . A more detailed understanding of these materials will help in their targeted optimization for these tasks.
By L. Gallmann / K. J. Kessler (ETH Zurich) and J. Witt (MPSD).
[1] Z. Schumacher, S. A. Sato, A. Niedermayr, L. Gallmann, A. Rubio, U. Keller, "Ultrafast electron localization and screening in a transition metal dichalcogenide", Proc. Natl. Acad. Sci. 120(15), e2221725120 (2023), doi: 10.1073/pnas.2221725120
[2] M. Volkov, S. A. Sato, F. Schlaepfer, L. Kasmi, N. Hartmann, M. Lucchini, L. Gallmann, A. Rubio, U. Keller, "Attosecond screening dynamics mediated by electron-localization in transition metals", Nature Phys. 15, 1145-1149 (2019), doi: 10.1038/s41567-019-0602-9
[3] A. Niedermayr, M. Volkov, S. A. Sato, N. Hartmann, Z. Schumacher, S. Neb, A. Rubio, L. Gallmann, U. Keller, "Few-femtosecond dynamics of free-free opacity in optically heated metals", Phys. Rev. X 12, 021045 (2022), doi: 10.1103/PhysRevX.12.021045