
Photo-induced dynamics of correlated systems
In complex materials, phases such as magnetism and superconductivity arise from the collective interplay of many degrees of freedom. Dynamically controlling this collective behavior would provide intriguing possibilities to manipulate complex material properties on ultra-fast timescales, possibly allowing for technological applications such as ultra-fast magnetic storage or femtosecond optical switches. In our group, we aim to compute the time-resolved photo-induced dynamics in complex correlated systems from microscopic theory. Below is an incomplete selection of examples:
Ultra-fast control of magnetic interactions

Properties of magnetic states are governed by the exchange interaction J, which can be of the order 1000 Tesla in condensed matter systems. In contrast, magnetic fields in the laboratory typically do not exceed several Tesla. This also sets a limit for the speed of magnetic hard-discs, as long as the time needed to reverse a magnetic bit is set by the field of the write head. Manipulating J would be an intriguing way to push the speed limits of magnetism control.
Because the exchange interaction emerges from electronic properties, it can be modified in an ultra-fast way. This could be demonstrated in recent studies for a laser-excited antiferromagnetic Mott insulator: Adding mobile carriers (photo-doping) weakens the exchange. The same effect would be reached by chemical doping, but photo-doping allows this change to happen within the electronic relaxation time time, i.e., quasi instantaneous on the timescale of the spin-dynamics. In a complementary approach, we showed that the exchange interaction can also be controlled by coherent light-dressing of the electronic states. In this way, the exchange interaction can be switched reversibly with the laser light, without the irreversible electronic excitation. In the extreme case, even the sign can be changed from ferromagnetic to antiferromagnetic. In future studies we plan to investigate similar concepts to control the emergent interactions between magnetic, orbitally ordered, charge ordered, or superconducting phases, to see how these states can be controlled with light.
For more information, see:
- J.H. Mentink, K. Balzer, and M. Eckstein, Nature Comm., 6, 6708 (2015)
- J.H. Mentink and M. Eckstein, Physical Review Letters, 113, 057201 (2014)
Correlated surfaces and heterostructures
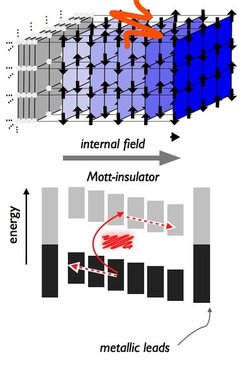
Artificially designed hetero-structures provide an important route to control correlations in functional devices. To study the dynamics in such systems we have extended the nonequilibrium DMFT formalism to layered inhomogeneous systems.
As a first application, we consider a stack of several layers of a Mott insulating material, sandwitched between metallic leads. In a material of polar layers, there can be a strong internal electric field between the layers, which can be beneficial for separation of photo-induced carriers in photovoltaic devices. Our simulations show the somewhat counterintuitive result that large fields can be detrimental for efficient carrier separation: One finds ultrafast separation of photo-excited carriers within femtoseconds for an antiferromagnetic background, but not for the paramagnet. In the strongly non-linear transport regime, carriers need an efficient channel to dissipate their potential energy as they diffuse along the direction of the field. This energy sink is provided by antiferromagnetic correlations.
For more information:
- M. Eckstein and Ph. Werner, Physical Review Letters, 113, 076405 (2014)
Polaron formation in nonequilibrium
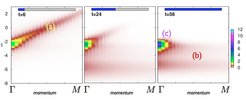
An important aspect of light-induced dynamics is the interplay of lattice and electrons. When mobile electrons in a material interact strongly with the atoms in the crystal lattice, they can form a new entity, a polaron, in which the electron along a distortion of the surrounding lattice. This effect drastically reduces the mobility of the particles and thus has profound influences on the conductivity. The question of how an initially free electron transforms into a polaron on pico or femtosecond timescales is of central importance for the understanding of laser-induced metallic states, which themselves often live only a picosecond.
We have provided an exact solution of the polaron formation process for high-dimensional bulk lattices, based on the recently developed nonequilibrium dynamical mean-field theory. The figure shows computed snapshots of the time- and momentum resolve photoemission spectrum. The formation of heavy polarons is indicated by a flat-band feature. However, an important observation is trapped in an excited state with very different properties for rather long times: Polaronic states coexist with delocalized (mobile) electrons (indicated by a momentum dependent peak at the Gamma point in the photoemission spectrum), which are prevented from polaron formation by an energy barrier. The existence of delocalized, conducting, states suggest that in a material with strong electron phonon interaction, properties of photo-induced metals may be manipulated by external fields in a controlled manner.
- Sharareh Sayyad and M. Eckstein, Physical Review B, 91, 104301 (2015)