Telling mirror molecules apart
A novel technique reliably tells left-handed from right-handed variant of a compound
The chemistry of life is built on left-handed and right-handed molecules that can have completely different functions. A novel technique, developed by researchers from the US and Germany, can reliably tell these mirror molecules apart. The new method can in principle even detect these so-called enantiomers in mixtures of substances. The procedure also holds promise for the development of a technique to separate left- from right-handed variants of a molecule, reports the international research team, which also includes Melanie Schnell of the Center for Free Electron Laser Science CFEL. CFEL is a joint venture of DESY, the Max Planck Society and the University of Hamburg.
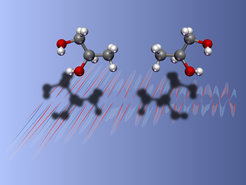
Many chemical compounds exist in two variants, called enantiomers. These consist of the same elements but act as mirror images of each other. In reference to our hands, which are also mirror images, such molecules are called chiral, after the ancient Greek word for hand, cheiros. "Distinguishing the two variants of a chiral compound is one of the most difficult, yet most important tasks in analytical chemistry," says David Patterson from the University of Harvard, who is the first author of the "Nature" paper. In biology, but also in many chemical reactions, the chirality of a molecule plays an important role. The chemistry of life, for instance, builds almost exclusively on left-handed amino acids and right-handed sugar molecules. Why and how nature does this, is a largely unanswered question.
Chemical syntheses often produce both variants (enantiomers) of a compound in equal amounts. "The 'wrong' sort of a compound can function completely differently in an organism," explains Melanie Schnell, who leads an independent Max Planck Research Group for Structure and Dynamics of Molecules at CFEL. "In the best case it is just ineffective. In the worst case it is toxic." Especially the pharmaceutical industry is hugely interested in the production of pure enantiomers, and the development and improvement of methods to synthesise or later enrich a certain enantiomer of some active substances is an active and broad field of research.
Enantiomers can be distinguished by their dipole moments
But already just telling apart the two variants of a chiral molecule is generally no easy job. The enantiomers share almost all physical characteristics. The easiest way to discern between them is to shine linear polarised light through them. While one variant will turn the plane of polarisation to the left, the other will turn it to the right. That's why the enantiomers are called laevorotatory and dextrorotatory. But especially in mixtures of enantiomers and in mixtures of different substances, these effects are usually small, and they can only be seen in liquid samples.
In contrast, the method developed by David Patterson, Melanie Schnell and John Doyle uses a different characteristic of the enantiomers, called the dipole moment. This describes the interaction of a molecule with an external electric field. Although the dipole moment components of both enantiomers are always of the same magnitude, they differ in sign - which is a direct consequence of their mirror-image construction. Thus, measuring the sign of the dipole moment components reveals the type of the enantiomer.
The team used microwaves to probe the dipole moments of enantiomers. The sample is fed as a gas into a cold chamber and cooled down to minus 266 degrees Celsius. Many biologically relevant compounds as well as many of those used in the chemical industry can be brought into the gas phase. The cold gas interacts with a fine-tuned microwave field that excites distinct rotations of the molecules. While rotating, the molecules themselves emit microwaves that can be recorded. The phase of this radiation reveals the enantiomer type: When the radiation of left-handed molecules has reached the positive maximum, the radiation of right-handed molecules is at its negative maximum.
Further development of the method will enable separation of enantiomers
The researchers tested their method using the organic compound 1,2-propanediol, which can be bought as pure enantiomers. The technique could not only reliably differentiate between the two variants, but also tell the mixing ratio of a mixture of enantiomers. The microwave frequency can be extremely fine tuned to the desired rotational state of a molecule. This way, the technique can also probe mixtures of substances. "We can soon measure mixtures of different compounds and determine the enantiomer ratios of each," explains Schnell. In a next step the researchers plan to apply the technique in a broadband spectrometer at CFEL that could then measure the enantiomer ratios in mixtures of substances.
"In the longer run, the method opens the exciting perspective to develop a technique for separating enantiomers," explains Doyle. To this end, one enantiomer could be excited with a laser. Then, another laser that acts differently on excited molecules than on not excited molecules can be used to separate both enantiomers. These laser pulses could be repeatedly applied to a room-temperature vapour in a closed cell. Typically, only one in ten thousand molecules would be in the desired rotational state at any moment, but a significant enantiomeric excess could be rapidly accumulated.
DESY/PH