Profiling X-ray free-electron laser pulses
Physicists demonstrate crucial method for monitoring ultra short X-ray pulses
With their ultra short X-ray flashes, free-electron lasers offer the opportunity to film chemical reactions or atoms in motion. However, for this super slow motion the arrival time and the temporal profile of the pulses must be precisely known. An international team of scientists has now developed a measurement technique that provides complete temporal characterization of individual FEL (free-electron laser) pulses at DESY's soft-X-ray free-electron laser FLASH. The team, led by Adrian Cavalieri from the Max Planck Research Department for Structural Dynamics at the Center for Free-Electron Laser Science (CFEL) in Hamburg, was able to measure the temporal profile of each X-ray pulse with femtosecond precision (a femtosecond is a quadrillionth of a second). Their technique can be implemented at any of the world's X-ray free-electron lasers, ultimately allowing for most effective utilization of these sources. The results are published in the current issue of the scientific journal Nature Photonics.
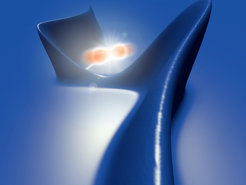
X-ray pulses delivered by free-electron lasers provide unique research opportunities, because the pulses are ultra-intense and ultra-short. At FELs trillions of X-ray photons are packed within a single burst – or pulse – which lasts for only several tens of femtoseconds, or even less. However, the precise arrival time and even the temporal profile of the FEL pulse can change dramatically from one pulse to the next.Therefore, to use the FEL to “film” ultrafast dynamical processes, the arrival time of each pulse must be measured to reorder the individual frames or snapshots captured with each individual FEL pulse.
Provided with accurate timing information, femtosecond FEL X-ray pulses are short enough to study atoms in motion, chemical reactions, and phase transitions in materials with atomic resolution on the femtosecond timescale.
With simultaneous measurement of the FEL X-ray pulse profile, it will be possible to go even further, to explore processes that evolve within the X-ray exposure. On these timescales the motion of electrons and electronic state dynamics become significant. Electronic dynamics drive damage processes in biomolecules, which may destroy them before they can be recorded in a crystal clear image.
For their measurements, the team which includes scientists from CFEL, DESY, European XFEL, Dublin City University, University of Hamburg and SLAC adapted a technique used in attosecond science called “photoelectron streaking” (an attosecond is a thousandth of a femtosecond). With this, the temporal profiles of varying light signals can be recorded. By taking advantage of the ultra-high intensities available at FELs the researchers were able to perform the streaking measurement on a single-shot basis at FLASH. For this, the X-ray flashes were shot through neon gas on their way to their target. Each pulse ejects a burst of photoelectrons from the noble gas. The temporal profile of the photoelectrons is a replica of the FEL pulse that ejected them.
With an electromagnetic terahertz (THz) field, the photoelectrons are accelerated or decelerated, depending on the exact instant of their ejection.
The strength of this effect is measured using time-of-flight spectroscopy. If the exact shape of the terahertz pulse is known, combining this information reveals the temporal profile and arrival time of the individual X-ray pulses with a precision of about 5 femtoseconds.
“Simultaneous measurement of the arrival time and pulse profile, independent of all other FEL parameters, is the key to this technique,” explains Cavalieri, who is a professor at the University of Hamburg and a group leader in the Max Planck Research Department for Structural Dynamics (MPSD). Until now, no other measurement has provided this complete timing information – yet it is exactly this information that will be crucial for future application of these unique X-ray light sources.
The FEL pulse characterization measurements presented by the team are made without affecting the FEL beam – only a negligible number of photons are lost for creating photoelectrons. Therefore, they can be applied in any experiment at almost any wavelength, for example at the European XFEL which is currently under construction in Northern Germany. In the immediate future, laser-driven THz streaking will be used to monitor and maintain the FEL pulse duration at FLASH to study a wide variety of atomic, molecular and solid-state systems. For further experiments, the researchers plan to use these high precision measurements as critical feedback for tailoring and manipulating the X-ray pulse profile.