New ultrathin water layer method puts hydrogen bonds on show in TEM
MPSD researchers develop a new method for water diffraction in transmission electron microscopes. Their work has appeared as a featured article in the Journal of Chemical Physics.
H2O: Two Hydrogen atoms attached to an oxygen. This is water – a deceptively simple molecule involved in complex interactions with other molecules and, intriguingly, itself. The reason for this complexity can be summed up in one concept: the hydrogen bond. These bonds are strong connections and give liquid water a structure resembling a disordered crystal lattice. This structure produces many unique properties in water, from its relatively high boiling point despite a low molecular mass to the fact that ice floats on water and also its ability to at least partly dissolve a great majority of compounds. All of this has made water the primary milieu in which life occurs.
Scientists quantify water and other liquid structures through the average number of atoms bonded to a central atom, known as the coordination number. Electron scattering measurements, which can be conveniently performed using widely available transmission electron microscopes (TEMs), are a particularly good way to determine this number. However, this poses major experimental challenges, because extremely thin layers of liquid must be held in the high vacuum of the TEM column.
“Water diffraction in a TEM has been done decades ago, but those researchers could only keep the water stable for a few seconds before it evaporated. That made it impossible to obtain high-quality data,” explains Sana Azim, a former PhD student at the IMPRS-UFAST graduate school. Together with a team of researchers in R. J. Dwayne Miller’s group at the MPSD and other collaborators, she has tackled this key problem by developing a pioneering device to obtain indefinitely stable layers of water only about 100 nanometers thick.
The ultrathin water layer in the TEM is held between two electron transparent silicon nitride window structures, separated by a 10-micrometer spacer and equipped with flow channels for circulating humid air. Notably, this method makes it possible to investigate water at near-ambient temperature and pressure.
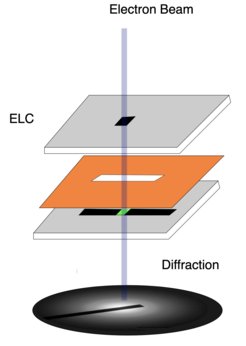
As so often, though, solving one problem creates another: Electrons scattering from the silicon nitride windows obscure the desired signal from the enclosed liquid layer. This effect needed to be filtered out. “By combining measurements from an empty and a full silicon nitride cell, we can disentangle the different contributions and focus only on the water content,” explains former MPSD postdoc Michiel de Kock, who developed the data analysis method. It is described in the researchers’ recent paper which has just appeared as a featured article in the Journal of Chemical Physics.
Essentially, de Kock’s method compensates for the electron scattering in the silicon nitride window and / or the water layer occurring more than once.
“It worked out really well,” adds Azim. “We were able to determine the total number of hydrogen bonds per water molecule as five from the calculated coordination number. This indicates a tetrahedral structure where the four hydrogen bonds present contribute to the structure. The fifth bond may either be weakly attached or not at all attached to the central molecule and facilitates the reorganization of the hydrogen bond network by reducing the energy barrier for translational and rotational motions.”
“We have managed to show that high structural detail can be extracted from water despite large background scattering from encapsulating layers,” says co-author and MPSD subgroup leader Günther Kassier. This makes the work relevant for other exciting fields as well: “Think of all the complex interactions occurring in and around water, such as chemical and biological reactions, structural intricacies resulting from solutes or interface effects at the solid-liquid or liquid-gas boundary.”