A first hint of superconductivity at room temperature
Scientists of the Max Plank Institute for the Structure and Dynamics of Matter have turned a normal insulating crystal into a ‘transient’ superconductor even at room temperature, using a flash of infrared light. The superconducting state survived only for a couple of picoseconds (trillionths of a second), but the findings may aid the quest for higher temperature superconductors, as the team of scientists including Wanzheng Hu, Daniele Nicoletti, Cassi Hunt and Stefan Kaiser led by Andrea Cavalleri from the Max Planck Institute for the Structure and Dynamics of Matter reports in the scientific journal Nature Materials.
Most materials heat up when you run a current through them. This is how hair dryers and curling irons work, and why your laptop will warm your lap while you use it. This heating is due to resistance in the material. Microscopically, what happens is that electrons bounce off of impurities in the material. The electrons ricochet around, which causes the atoms in the material to move and you feel this motion as heat. Even good conductors like electrical lines lose roughly 6% of the power they transmit due to resistive losses, so developing a low or zero resistance material has immediate practical benefit.
Superconductors show exactly zero electrical resistance, meaning that they can carry a constant current without any losses. Unfortunately, this phenomenon typically happens at very low temperatures: the so-called “critical temperature” below which superconductivity appears can only be reached by cooling these materials with liquid nitrogen (-200 °C) or even liquid helium (-270 °C). Many common materials like lead and mercury become superconductors at these temperatures. Aluminum only becomes a superconductor at temperatures colder than space!
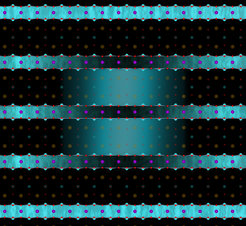
The “high temperature superconductors” are man-made materials with complex crystal structures that can superconduct at higher temperatures, but even the best must be cooled below -140 °C. We understand how low temperature superconductors like lead work, but the origin of superconductivity in high temperature materials is still a mystery. We know theoretically that the origin must be different from the low temperature materials, and the hope is that figuring out how they function will help us make new materials that superconduct even at room temperature.
Scientists have figured out some important clues. We know that, like low temperature superconductors, the electrons in man-made superconductors attract each other, forming pairs. (This is very strange in itself, because electrons normally repel each other strongly.) These pairs can move through the material as though they do not see any impurities. Through a quantum mechanical process called Josephson tunneling, they can also hop across small non-superconducting gaps. The cuprates are a group of high temperature superconductors that naturally have 2D superconducting planes, made of copper and oxygen atoms, separated by a gap. Hopping across the planes allows the material to act as a full, 3D superconductor.
Yttrium barium copper oxide (YBCO) is one of the most famous cuprate superconductors, with a critical temperature as high as -180 °C. Its crystal structure consists of stacks of two closely spaced copper-oxygen planes. Previous studies have shown that some kind of superconducting-like behavior is present far above the critical temperature in YBCO. Most likely, pairs of electrons can already hop between the closely-spaced copper oxygen layers, but not across the large distance to the next bilayer unit. This coupling over large distances sets in only at the critical temperature, when the material becomes a 3-dimensional superconductor.
“Our goal is to use light pulses to stimulate the electron pairs to tunnel freely between all layers at higher temperatures, thus effectively increasing the critical temperature,” explains Hu.
Method
The researchers use mid-infrared pulses to drive atomic motion in the YBCO crystal. Specifically, we target oxygen atoms that sit in the gap between pairs of copper-oxygen planes. The distance between these oxygen atoms and the planes has been found to be directly related to the critical temperature. We aim to modify the electron transport and possibly increase the critical temperature of YBCO.
Findings
After exciting the YBCO lattice, we find that the transport of electrons between copper-oxygen planes appears to be strongly modified. Our results suggest that the mid-infrared light causes the material to behave like a 3D superconductor even far above the critical temperature. We investigated two cases:
(1) Below the critical temperature, when the material is superconducting, the light appears to enhance superconductivity. We see evidence in the optical conductivity response of enhanced Josephson tunneling between bilayers—more hopping across the gap between pairs of planes. This corresponds to more pairs of electrons contributing to the zero resistance state. This enhancement is compensated by less hopping within the pairs of planes. This means we are probably not creating new pairs, but instead changing the behavior of the pairs that are already there in a way that helps the superconducting state.
(2) Above the critical temperature, where the material is not a superconductor, the light appears to create a superconducting channel perpendicular to the Copper oxide planes. Rather than creating superconducting pairs, our results support the idea that these pairs are already present and already tunneling across the closely-spaced planes in the bilayer. The crystal excitation allows the pairs to hop across the space between bilayers. The optical conductivity of the light-induced state above the critical temperature is very similar to the conductivity in the superconducting state below the critical temperature without light excitation. Surprisingly, this “transient” superconducting state can be induced even up to room temperature.
Current Limits and Open Questions
(1) The light only transforms a fraction of the entire material (< 20%). Can one generate a homogeneous superconductor with light?
(2) The mid-infrared light is delivered as a pulse that lasts less than half a picosecond, and the entire effect lasts only a few picoseconds. Is there a way to extend the lifetime of the light-induced state?
(3) How does exciting the oxygen atom between copper-oxygen planes promote superconductivity? Can we develop a material that has the same properties, but without requiring light excitation—a true room temperature superconductor?