Catching Hund’s Rule in action
An atomic perspective on electron spin effects on spatial arrangement of matter
Electron spin is one of the most fundamental quantities defining the properties of molecules. Physics and chemistry students are routinely taught the Pauli exclusion principle and Hund’s rules at the undergraduate level. The Pauli exclusion principle mathematically ensures that no two electrons can occupy the same space at the same time much like two people cannot occupy the same chair at the same time.
Electrons are referred to as fermions and occupy space; the exact distribution is determined by electron-electron repulsion forces. Hund’s rule is related to the intrinsic properties of electron spin associated with the electrons’ motion about the nucleus. It is one of the most exotic properties of matter. Electron spin can only be understood by treating the relativistic aspects of electron motion that lead to an effective magnetic dipole moment. It is the forces between the intrinsic electron spins that further change the spatial arrangement of electrons.
This is an exceedingly complex concept, easy to formulate, difficult to understand physically. Hund’s rule was formulated on empirical evidence which states that the lowest energy electron distribution, in the case of degenerate (same energy) electronic levels, is for the electrons to occupy different spatial orbitals or arrangements with their spins parallel. Fundamentally, it is the additional forces of the magnetic dipoles associated with the electron motion that make the electrons seek different spatial distributions.
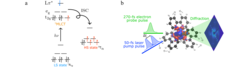
Even at the high school level, we are taught to dutifully put electrons as unpaired parallel spins into degenerate orbitals to predict the lowest energy state or electron configurations. We can make powerful predictions on whether one observes magnetism or not based on this simple rule. Could we ever directly observe the physics behind Hund’s rule?
Questions remain on how spin state changes directly affect molecular structures and dynamics. Until now, ‘wiggly arrows’ have been used to depict this nonradiative relaxation to the lowest energy spin state. The recent study by an international research team from Germany, Canada, Poland, and Lebanon offers a detailed understanding on how changes in spin states affect the nuclear configuration as a probe of the change in electron distribution by directly observing the atomic motions and change in the molecular structure, driven by change in electron spin. In addition, this work establishes a detailed picture of the causal relationship between the electronic spin dynamics of the molecular system and the coordinating ligands (see movie).
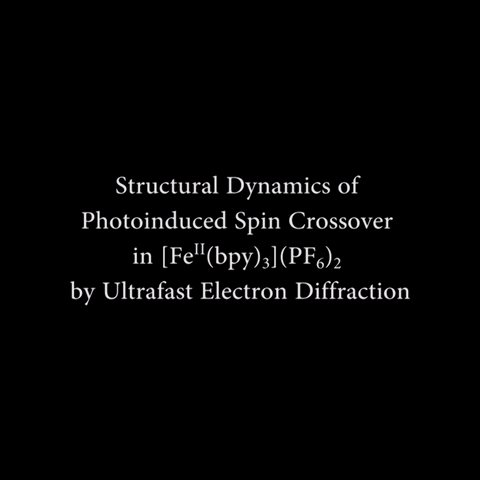
The team’s paper, now published in Nature Communications, discusses the coupling between spin and nuclear degrees of freedom at the atomic level by comparing the results of two different spin crossover (SCO) compounds, rationalizing these differences in terms of the nonradiative relaxation processes and ligand-induced symmetry effects. Lead author Dr. Yifeng Jiang, now a scientist at the European XFEL and a postdoc at the MPSD at the time, says: “We uncover the key structural modes, ultrafast Fe–N bond elongations coupled with ligand motions, that define the effective reaction coordinate to fully capture the relevant molecular reorganization stabilizing the spin state.”
R. J. Dwayne Miller, the head of the research team and principal investigator of this work, states: “Electron spin is one of the most profound concepts of matter, which we can only understand through a relativistic treatment of electron motion. We arrive at seemingly simple rules and pictures for how electrons arrange in space, depending on their relative spin. These simple relations are really a reflection of the intrinsic properties of the electron and how many body effects lead to the wonders of nature. We can now directly observe the forces involved in electron spin transitions - to give a direct observation of Hund’s rule in action.”
These results present new inroads for the control of molecular properties through spin. Chemistry may catch up with physics in fully exploiting spin with these new insights. For example, several SCO-active transition metal complexes have been studied as prototypes for light harvesting since they can convert light to chemical energy via photoinduced charge separation. Ligands, binding to the central metal atom, are widely used to fine-tune the relative energy of the excited states visa ligand-field effects. Chemical tailoring of the ligands to gain control over these spin dynamics and material properties has become a current research focus, which further illustrates the importance of understanding nuclear reorganization processes that stabilize spin transitions.