A remote control for functional materials
When mid-infrared laser pulses hit certain complex materials, their fundamental properties can change in astonishing ways. They may become magnetic, ferroelectric or begin to carry electrical currents without any resistance. A key ingredient of these phenomena is the efficient excitation of the collective atomic motions of the solids, known as phonons. Typically, the periodically aligned atoms of the crystal lattice vibrate only around their equilibrium positions. However, in the case of very intense excitation fields, they will do so at colossal amplitudes – with the consequence of displacing certain atoms away from their equilibrium positions and through this way changing electronic or magnetic functionality. This phenomenon came to be known as nonlinear phononics.
So far, nonlinear phononics has been thought to occur only within the small region of the crystal where the mid-infrared light pulse is present. Now researchers in Hamburg have discovered that the ferroelectric polarization of lithium niobate (LiNbO3) even changes in areas well away from the direct ‘hit’ of the laser pulse, with the polarization reversal occurring throughout the entire crystal. The team’s study of this hitherto unknown phenomenon - called nonlocal nonlinear phononics - has been published in Nature Physics.
Ferroelectric materials such as LiNbO3 possess a static electric polarization generated by lines of positive and negative charge that can be switched with an electric field. This unique property makes these materials the basic building block of many modern electronic components in smartphones, laptops and ultrasound imaging devices. Using laser light to change the ferroelectric polarization is a new approach that allows for extremely fast processes which would be a key step in the development of highly efficient ultrafast optical switches for new devices.
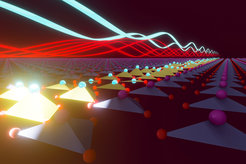
The researchers in Andrea Cavalleri’s group at the Max Planck Institute for the Structure and Dynamics (MPSD) used mid-infrared pulses to excite the surface of a LiNbO3 crystal, launching a strong vibration throughout a region that spans a depth of 3 micrometers from the crystal surface. Then, they used a technique called femtosecond stimulated Raman scattering to measure ultrafast changes of the ferroelectric polarization throughout the complete 50 micrometer crystal thickness. The measurements revealed that light pulses with a very high energy density cause the ferroelectric polarization to reverse throughout the entire crystal. By using computational methods to simulate the effects of nonlinear phononics in LiNbO3, the authors found that strong polarization waves called polaritons emerge from the small volume traversed by the light pulse and move throughout the remaining depth of the crystal. These polariton waves are believed to play a significant role in altering the ferroelectric polarization throughout the sections of the crystal that are untouched by the light pulse.
The results reported by Henstridge et al. add an exciting new piece to the elusive puzzle of ultrafast ferroelectricity, the understanding of which can lead to new device components such as sustainable optical switches. More broadly, this work opens an enormous question concerning whether past and future systems driven by nonlinear phononics can exhibit a similar type of nonlocal character. The ability to manipulate functional properties at a distance could expand the realm of possibilities for incorporating nonlinear phononics into integrated devices and other complex materials, opening new avenues for controlling systems with light.
This research received support from the Deutsche Forschungsgemeinschaft via the Cluster of Excellence CUI : Advanced Imaging of Matter.