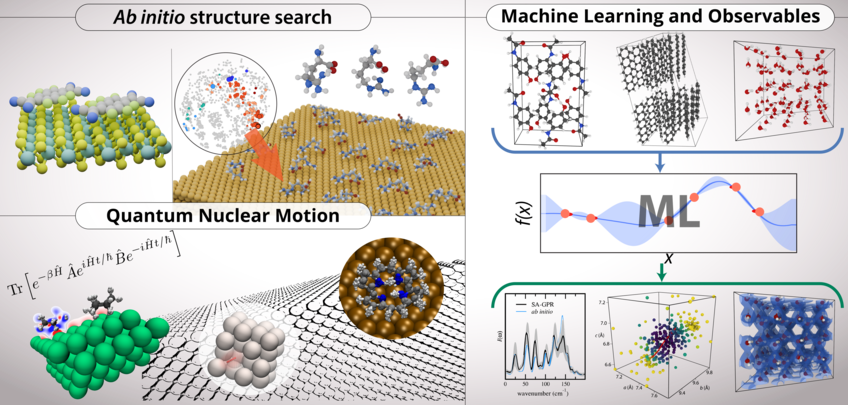
Research
The research in our group focuses on the development of practical methodology that can unravel the electronic and nuclear properties of complex materials. We work with applications that can be directly connected to experiments. In the following, we give more details about the current research fronts.
Machine Learning Nuclear and Electronic Structure Properties
The increased computational cost of accounting for nuclear fluctuations and dynamics with ab initio potentials calls for the development of techniques that can decrease this cost without sacrificing the accuracy of simulations. Machine-learned potentials allow the calculation of longer time scales and larger system sizes, but they do not give direct access to electronic structure properties. We work on the develpment of machine-learning models targeted at electronic-structure observables in order to overcome this drawback. Recently, we extended a method based on symmetry-adapted Gaussian process regression algorithm to predict the electronic density (and properties derived from the density) of a wide variety of solids. This method accurately predicts the density of large supercells of, e.g., ice crystals, even when trained only on very small cells [1,2]. We have also employed different flavors of GPR to predict finite-temperature free energies of organic crystals that gives access to their lattice expansion [3]
[1] A. Lewis, A. Grisafi, M. Ceriotti, M. Rossi, Learning Electron Densities in the Condensed Phase, J. Chem. Theory Comput. DOI: 10.1021/acs.jctc.1c00576 (2021)
[2] Raimbault, N., A. Grisafi, M. Ceriotti, M. Rossi: Using Gaussian Process Regression to Simulate the Vibrational Raman Spectra of Molecular Crystals. New Journal of Physics 21,105001 (2019)
[3] M. Krynski and M. Rossi, Efficient Gaussian process regression for prediction of molecular crystals harmonic free energies, npj Computational Materials 7, 169 (2021)
Structure and Dynamics of Quantum Nuclei
The inclusion of nuclear zero-point-energy and tunneling effects in high-dimensional anharmonic systems represents a considerable challenge in atomistic simulations. We develop and optimize methodology in the realm of path-integral-based methods (path integral molecular dynamics and ring polymer instanton). These methods can give access to reaction rates and vibrational spectra including nuclear quantum effects and are fully applicable to high-dimensional anharmonic systems. With these techniques, we were able to resolve isotope effects in work-function changes in metal-organic interfaces and discover that surface fluctuations can enhance the intramolecular hydrogen tunneling rate by orders of magnitude for molecules adsorbed on metallic surfaces . Recently, we have extended the ring-polymer instanton method to include non-adiabatic effects that can be modelled as an electronic friction, which is especially relevant for adsorbates and impurities on metal surfaces or in bulk metals.
Nuclear quantum effects in dynamical observables
A few approximate methods based on path integral molecular dynamics for the inclusion of nuclear quantum effects on dynamical observables such as reaction rates, diffusion coefficients, and vibrational spectra have been proposed over the years, such as ring polymer molecular dynamics (RPMD) and centroid molecular dynamics (CMD). We augmented first augmented the RPMD method by attaching optimally damped white noise Langevin thermostats to the internal modes of the ring polymers, and thus coined this method thermostated ring polymer molecular dynamics (TRPMD) TRPMD is a method that heals the artificial resonances observed in vibrational spectra calculated with RPMD, that is more ergodic than RPMD, and allows the use of time steps for integrating the equations of motion that are much larger than the ones allowed by methods like CMD. It remains, however, just an approximation to quantum real time correlation functions, and inherits all other limitations of the original RPMD method. Nevertheless, it is a very good approximation especially when treating larger systems or approaching condensed phases – and surely the most efficient of its kind to be used together with ab initio methods. Recently we have further exploited the freedom in attaching different kinds of thermostats to the internal modes of the ring polymer and obtained much sharper vibrational spectra for molecules and low-temperature solid phases than with the original TRPMD. Developments in this area continue in our group.
Vibrational Spectroscopy
Complementary to IR spectroscopy, Raman spectroscopy can be used for a detailed structural characterization of nanostructures. With more recent experimental techniques that can enhance the Raman signal, like surface enhanced or tip enhanced Raman spectroscopy (SERS and TERS), and that can be conducted in very low temperature and ultra-high vacuum, as well as in solution, this technique has become a fundamental and versatile technique to obtain structural fingerprints. These spectroscopic techniques can probe molecular time scales of the order of femto- to picoseconds, which are within reach of current quantum mechanical techniques. The simulation of Raman spectroscopy from a first-principles perspective involves the calculation of the (zero-frequency) dipole polarizability tensor of a system from an electronic structure calculation. While it is possible to calculate such a quantity for smaller molecules with a finite differences approach, for larger and periodic systems density functional perturbation theory (DFPT) is the method of choice. In the all electron, numerical localized orbitals code FHI-aims, we have currently extended and optimized an all-electron, real-space-based DFPT implementation for the response to electric fields. Anharmonic Raman intensities can be calculated through the Fourier transform of time correlation functions involving the static polarizability tensor.
More generally, we develop simulation techniques that are able to account for anharmonic effects, nuclear quantum effects, and spatial localization in IR, Raman and sum frequency generation signals. We inverstigate spatially-localized Raman spectroscopy with realistic local electric fields, which can give access to atomic resolution of atomic motion of surface adsorbates. We also develop theory for the computation of vibrational sum frequency generation that can be applied to diverse materials by avoiding the need for an arbitrary molecular decomposition of the dipole moment or polarizability tensor of the system.
Structure search for flexible molecules at interfaces
Relatively reliable empirical potentials exist to describe of peptides in solution or isolated organic molecules, but in order to study their interactions with inorganic surfaces with predictive accuracy, it is mandatory to design new empirical models based on electronic structure calculations. For that, it is necessary to obtain a thorough exploration of the first-principles potential energy surface. A especially challenging aspect is to model reliably the surface-(bio)molecule interaction, accounting for the correct level alignments and charge rearrangements. We develop random structure search algorithms that act on internal degrees of freedom and optimize them for use with ab initio potential energy surfaces. In order to analyze and explore efficiently the free energy landscape, we exploit enhanced machine learning clustering techniques and venues for cheaper potential energy evaluation. Our goal is to reach longer time and length scales involved that allow the modeling of self-assembly of bio-organic molecules on surfaces.